Thursday 11th April 2024
At the last Meeting Dr.Joe Botting brought us all up to date with the new and exciting discovery of Burgess Shale type fauna in a quarry in mid-Wales. As often occurs, an exciting and important discovery comes just as work in an area is coming to an end. So it was with Joe and his wife Dr. Lucy Muir. They had been undertaking work in this area for 8 years but just as their work in this quarry was almost done they decided to take a last look at a very thin section they had not looked at previously. It was in this section that they made their highly important discovery.
Joe went on to explain that the middle Cambrian Burgess Shales as being one of the most important sites in the world in which many soft bodied animals are preserved, as carbon films, in very fine detail. In some cases not only is the soft tissue preserved but also internal structures, for example, neural tissue.This area and some other sites around the world, for example, The Chengjiang Biota have shown by how much life had evolved by the middle Cambrian. Not only do these sites show the fine details of the fauna but have also shown how markedly similar the fauna from all the sites actually is. It is from the Burgess Shale type formations that have shed light onto the Cambrian Explosion and the diversification of life. The end of the Cambrian sees little further diversification but is followed by the Great Ordovician Biodiversification Event leading to a large increase in biodiversity at the Family,Genus and Species level.
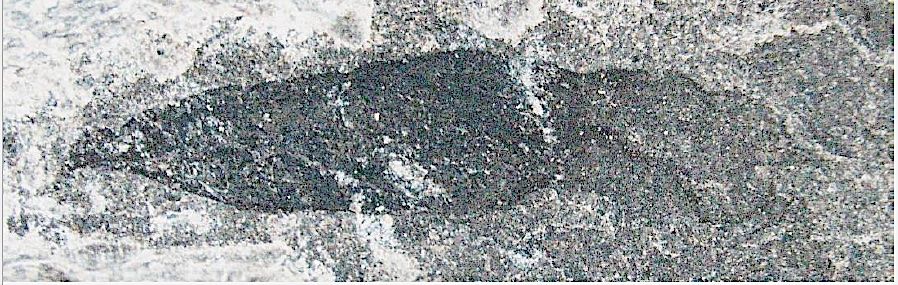
Fossil preserved as carbon film from Castle Bank By Joe Botting
He further explained that there are Ordovician Exceptional Fossil sites around the world but they are quite limited in one way or another when compared to the Burgess Shales, although there are two Ordovician Burgess Shale type areas, one at Afon Gam in north Wales and another at Fezouata in Morocco both from the early Ordovician. In contrast the Castle Bank fauna are from the middle Ordovician.
Castle Bank is situated within the northern part of the Builth Inlier. The stratigraphy here ranges from the Camnant mudstones through the Builth Volcanic Group to the Llanfawr Mudstones Formation. With fossils being found throughout.The area around Castle Bank is already well known for the fossil trilobites that can be found with the first trilobites being described from the area. Castle Bank itself is like any other farm quarry that can be found in the area with graptolitic shales and a few volcanic ash beds. But one section in this quarry was the one in which the soft bodied fauna was found. Like other fossils of the Burgess Shale type the fossils are preserved as carbon films and are very small!! The finds range from algae, hemichordates, sponges and many soft bodied animals that are still being identified. Joe and Lucy are working collaboratively with groups from other institutions on this task.
So in conclusion how good is castle Bank? Well, Joe suggests the following:
- At least 20 phyla (probably) - similar to Chengjiang.
- Nearly 200 species so far - similar to the Burgess Shales.
- Preservation of entire range of benthic organisms down to larger meiofauna.
- Some internal organ preservation and a lot of very soft and very fine tissue.
- Almost everything is new unlike the Cambrian Burgess Shale Type faunas which are similar to each other.
- Several major groups are the only fossil examples.
A fascinating talk on an equally fascinating discovery.
Sunday 10th March 2024
At the last meeting Chris Darmon and Colin Schofield gave a very informative talk on the North Atlantic Igneous Province (NAIP).
Chris explained that the NAIP is one of a number of what are known as Large Igneous Provinces ( LIP) which may be defined as “an intraplate magmatic event, with volumes of magma greater than 100,000 cubic kilometres". A couple of examples of other LIP’s are the Siberian Traps in Russia and the Deccan Traps in India. The NAIP is one of the larger LIP”s and is almost comparable with the Siberian Traps. It is centred on Iceland and occurs onshore in Greenland, the Faeroe Islands, the UK and Ireland and offshore surrounding these areas as well as the west coast of Norway.
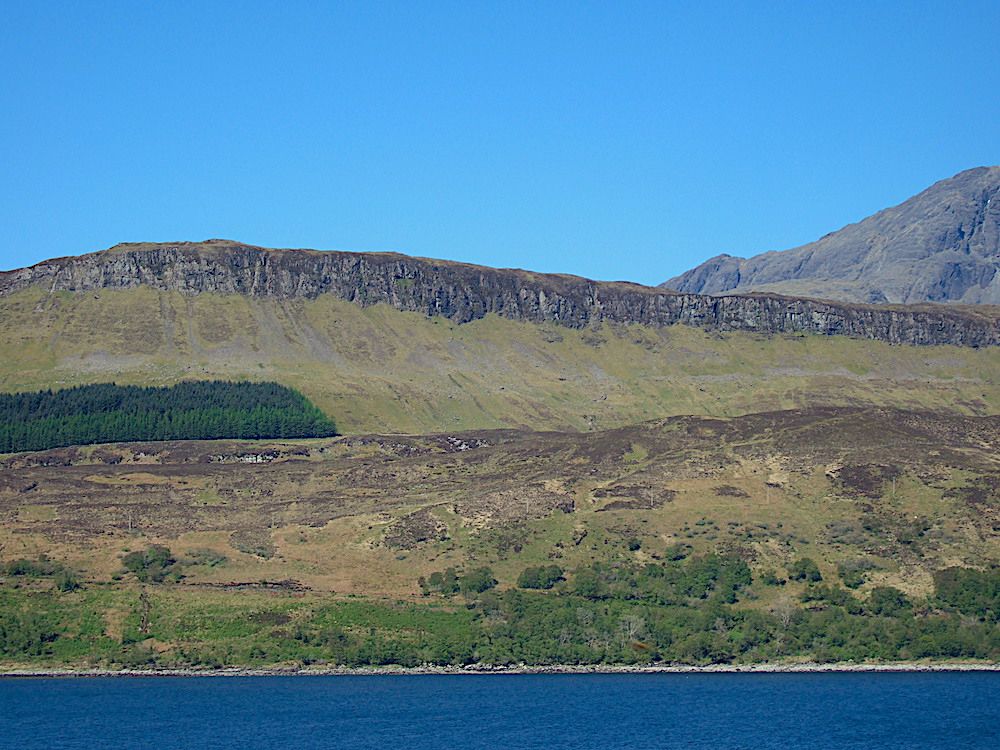
Edge of a lava flow along the West coast of Skye.photo By Chris Simpson MWGC
The NAIP is made up of flood basalts,sills and dykes which were produced by intense igneous activity occurring during the Palaeocene and early Eocene. The cause of this activity being continental separation during the opening of the Atlantic Ocean. Isotopic dating has been undertaken which indicates that the most active magmatic phase of the NAIP was between ca.60.5 and ca. 54.5 Ma (mid-Palaeocene to early Eocene). The volcanism lasted for about 11 million years although each individual eruption was for a much shorter duration of about 2-3 million years.
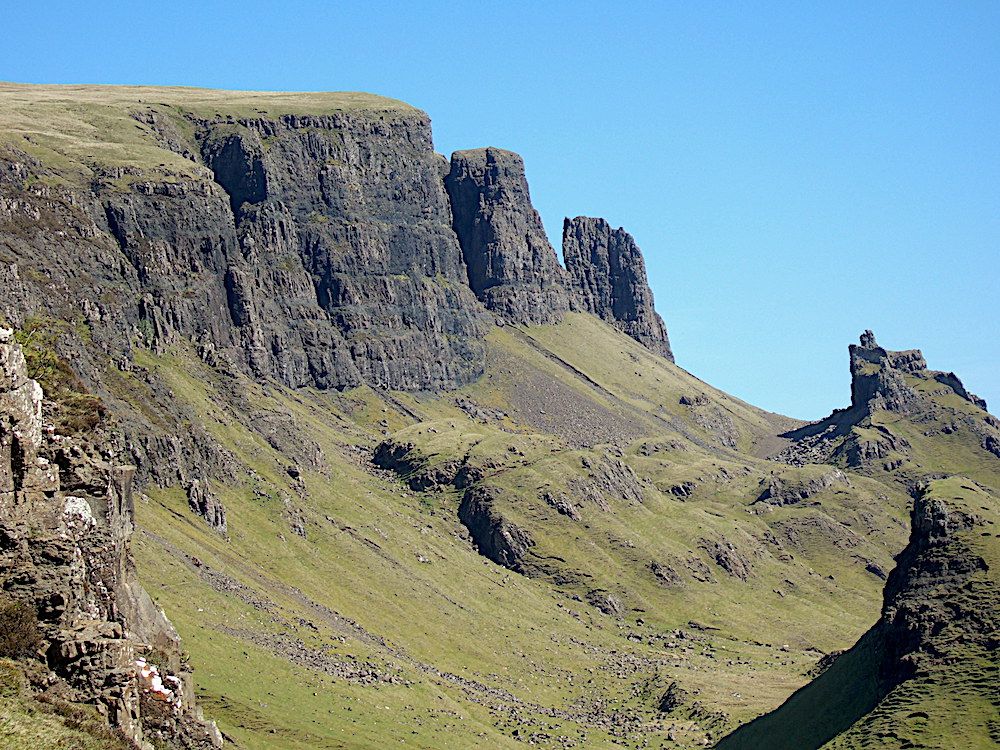
The edge of a lava flow in NE Skye.There has been a landslip with separation of the areas of rock at the right of the photo from the rest of the lava flow. Note how these separate areas have rotated anti-clockwise as they slipped down slope. The orientation of the successive flows is clearly dipping West compared to the roughly horizontal direction of the main flows on the left of the photo photo by Chris Simpson MWGC
The cause of this continental breakup and volcanism has been explained by the presence of a mantle plume which today is centred under Iceland but there is a lot of controversy in this area and other models have been suggested. There is a lot of ongoing research into this area so that a complete model should eventually be found.
The bulk of the talk actually centred on the British portion of the NAIP which is known as the British Palaeogene Igneous Province (BPIP). The area covered by the BPIP include the Isles of Skye; Rum, Mull; Eigg and Arran, the Ardnamurchan peninsula, outer Hebrides along with Lundy off the coast of Wales and the Mourne Mountains in Northern Ireland.
Prior to the opening of the Atlantic NW Scotland was joined to Greenland and the Canadian Shield but as the Atlantic opened NW Europe moved away from North America and this separation continues to the present day due to sea-floor spreading along the mid-Atlantic Ridge.
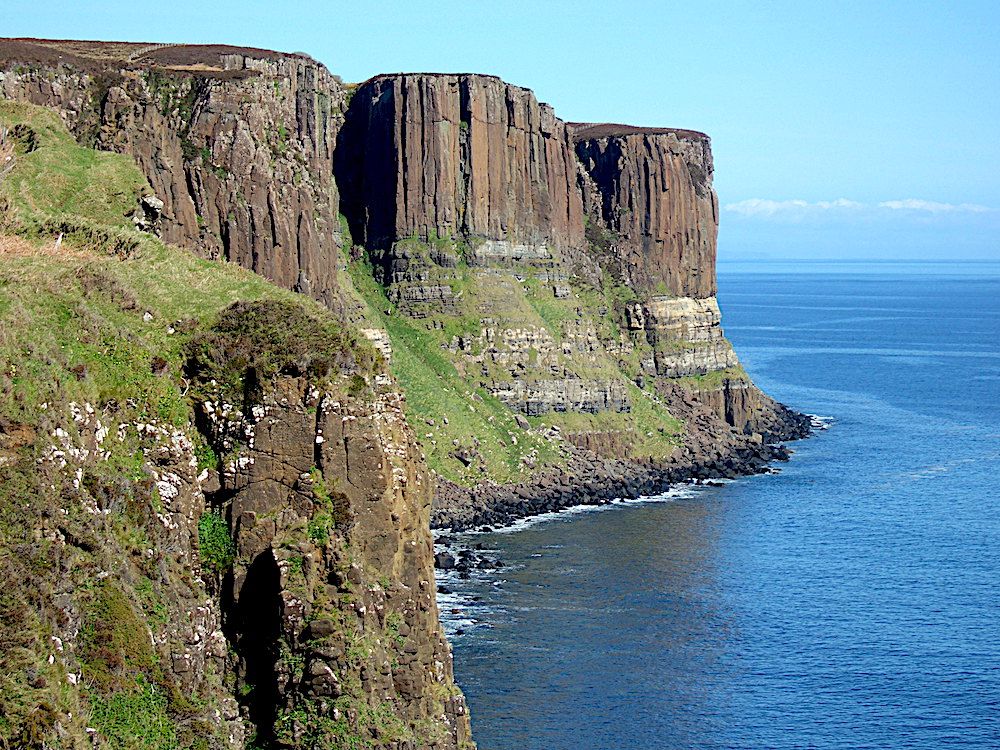
Kilt Rock - a sill displaying well-developed columns photo by Chris Simpson MWGC
As North America and Europe moved apart huge volumes of basaltic lava erupted from long narrow fissures on what is now the west coast of Scotland. In addition there were extensive dyke swarms extending to the Outer Hebrides, southern Scotland, north Yorkshire and parts of north Wales. There were intrusions of granite, gabbro, peridotite and other rock types. The basaltic lavas produced the stepped or “trap” landscape that may be seen on Skye. Lavas also produced the Giant’s Causway in Northern Ireland and Fingal’s cave on the Isle of Staffa.Gabbro and peridotite have given rise to the rugged mountain scenery of St Kilda,Skye (Cuillins) and Rhum. The less rugged, mountains of northern Arran and the Skye Red Hills are composed of granite.
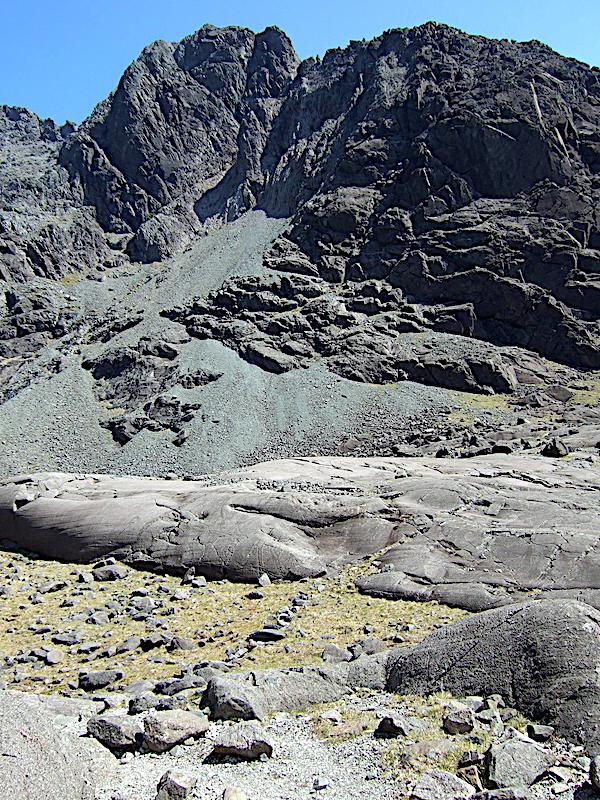
The Black Cuillins. This photo comes from about two-thirds of the way up. In the background are the tops of the Cuillins, black in colour and jagged in outline. In the foreground is smooth rock due to glaciation. It shows how high the glaciation came. Photo by Chris Simpson MWGC
Finally Chris highlighted the idea that the Palaeocene- Eocene Thermal Maximum (PETM), an event where there was a global increase of temperature between 5-8C can be linked to the NAIP producing changes in the earth’s carbon cycle and the increase in temperature. Work is still ongoing in this area.
In all a very instructive talk.
I
Friday 3rd November 2023
Quartz and other forms of silica by Bill Bagley (chair MWGC) 18th October 2023
All photos by Bill Bagley
Bill commenced the talk by showing that the vast majority of minerals that make up the rocks of the earth’s crust are silicate minerals such as quartz, feldspar, mica, amphibole, pyroxene, olivine, and many clay minerals. These silicate minerals account for 92% of the crust. Quartz forms 12% and it was on quartz that Bill concentrated the rest of the talk.
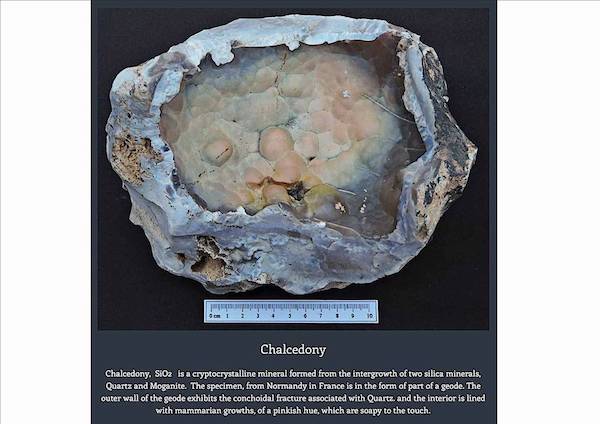
The building blocks of these minerals is the silica tetrahedron, a combination of four oxygen atoms and one silicon atom. These silica tetrahedral units can share oxygen atoms and be linked in a variety of ways, which results in different structures
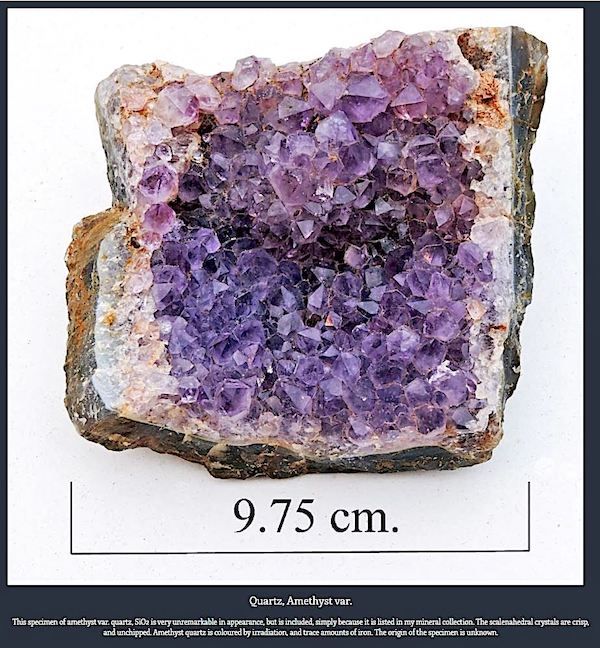
Quartz is composed of pure silica, SiO2 with the tetrahedra arranged in a three-dimensional framework. It is one of the most commonest minerals on earth. It can be found in many geological environments and is a constituent of many rocks. Quartz occurs in either a crystalline or cryptocrystalline form. The crystalline forms of quartz occur as distinct crystals for example amethyst, citrine and smokey quartz, whereas cryptocrystalline quartz is composed of inter-grown aggregates of microscopic or submicroscopic quartz crystals for example agate and onyx. The cryptocrystalline form of quartz may be divided into two general classes i.e. fibrous (eg. agate) and granular (eg.jasper)
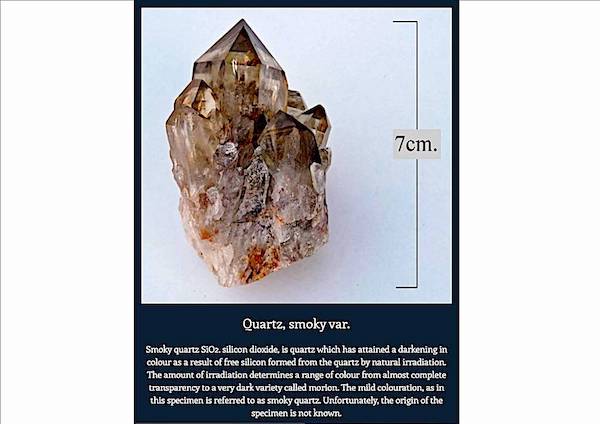
Pure quartz is usually clear or milky in colour but the presence of other elements in the lattice structure or structural change produces a myriad of colours. For example amethyst (violet/purple) is due to the presence of iron within the lattice; rose quartz due to the presence of titanium; smokey quartz due to radiation damage.
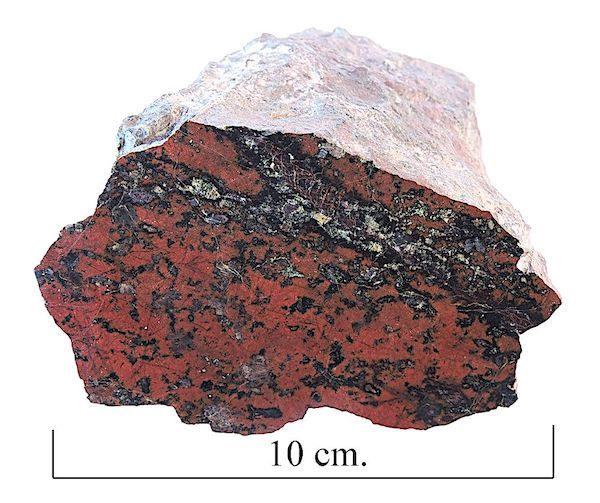
Jasper, Mookaite var.
Cryptocrystalline quartz is coloured similarly. For example agate which is characterised by concentric banding of colours is formed by silica-rich groundwater entering a void in a rock eventually forming a thin layer of chalcedony. This is then repeated over time. The differing colours are due to the presence of different impurities in each successive inflow of silica-rich groundwater. The most frequent impurities being iron oxide (red, brown or yellow); manganese oxide (purple or pink); chlorite (green); hematite (black).
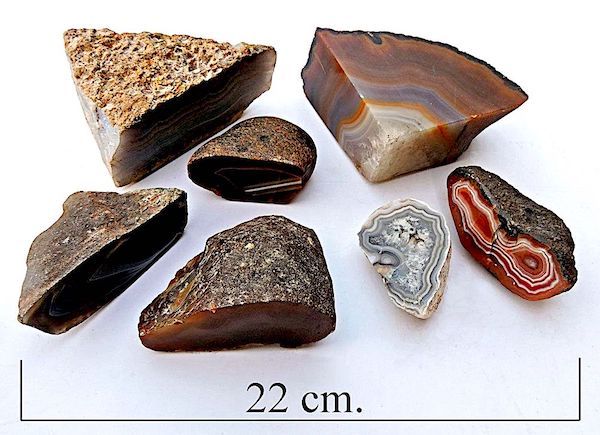
Australian agates.
Bill also explained some of the uses that silica can be put to both from the past and newer technologies.
The use of quartz dates back to the Palaeolithic where flint was used to make hand axes and scrapers.
In more modern times quartz is used in large quantities as it is an essential ingredient in concrete and mortar. Sandstone and quartzite are used in buildings and crushed in road and rail construction. Further, the properties of quartz means it has a wide variety of uses. Quartz has a hardness of 7 on the Mohs scale and may be used as an abrasive (eg. Glass grinding). It has a high melting point and can be used in the manufacture of refractory products like firebricks. Quartz crystals possess a property known as the piezoelectric effect. In other words it is the appearance of an electrical potential (a voltage) across the sides of a crystal when you subject it to mechanical stress. This for example is used in a quartz watch but there are many other uses in electronics.
A very interesting talk.
Monday 21st August 2023
Chris Simpson gave a talk summarising the main features of the geology of the Northwest Highlands of Scotland based on a 2022 guided tour with Chris Darmon and Colin Schofield.
The area is famous in geological circles because of the "Highlands Controversy" which divided 19th Century geologists – how could it be that old rocks should be found lying on top of younger rocks? The British Geological Survey sent two geologists to conduct an in-depth survey of the whole area and settle the controversy once and for all. Messrs Peach and Horne did just that. They found that older rocks were indeed emplaced overlying younger rocks as the result of large-scale thrust faults. Crustal compression during the Caledonian Orogeny had pushed millions of tons of rock over many miles to produce the Moine Thrust in the Northwest Highlands (and the Outer Islands Thrust Fault in the Outer Hebrides).
Chris also showed pictures of many of the other geological delights to be found in the Northwest Highlands.
(Summary and photos by Chris Simpson)
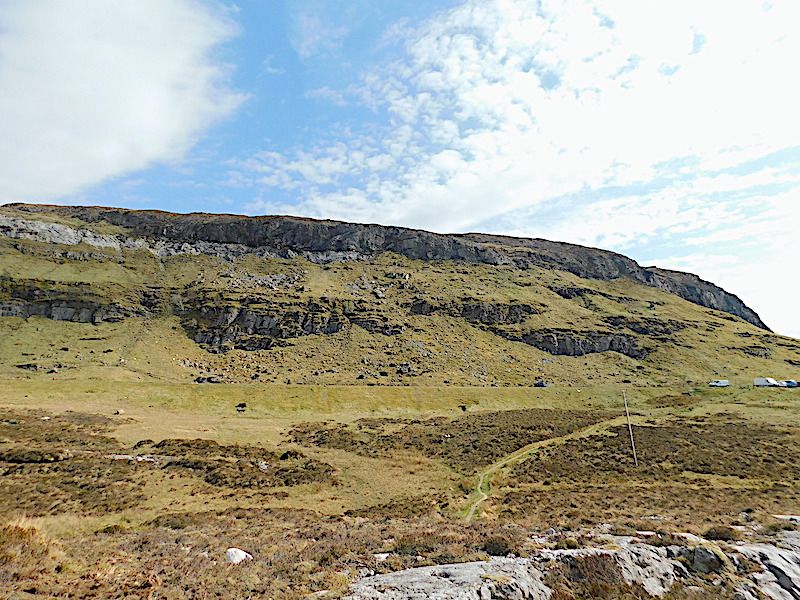
The Moine Thrust as seen at Knockan Crag. The dark layer at the top of the hillside is Moine schists which are thrust over pulverised Durness Limestones.
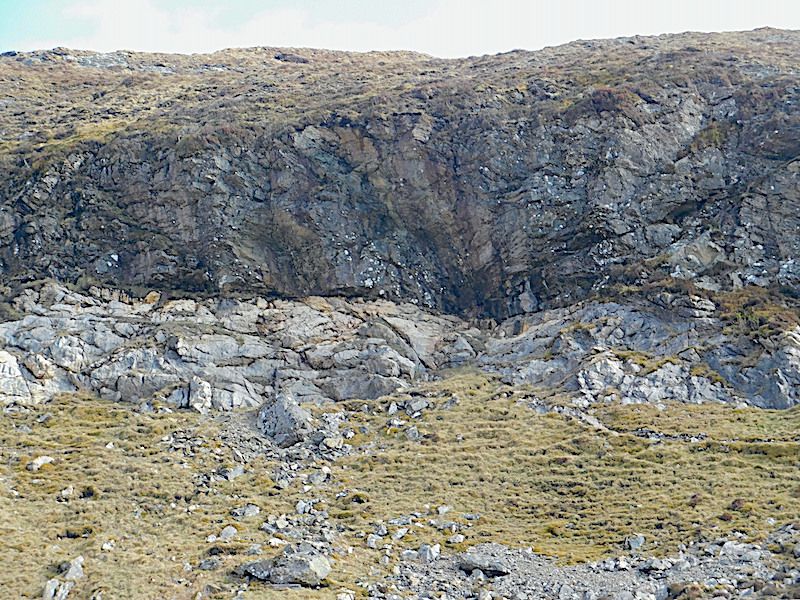
Close-up photograph showing contact between the Moine schists and Durness Limestone
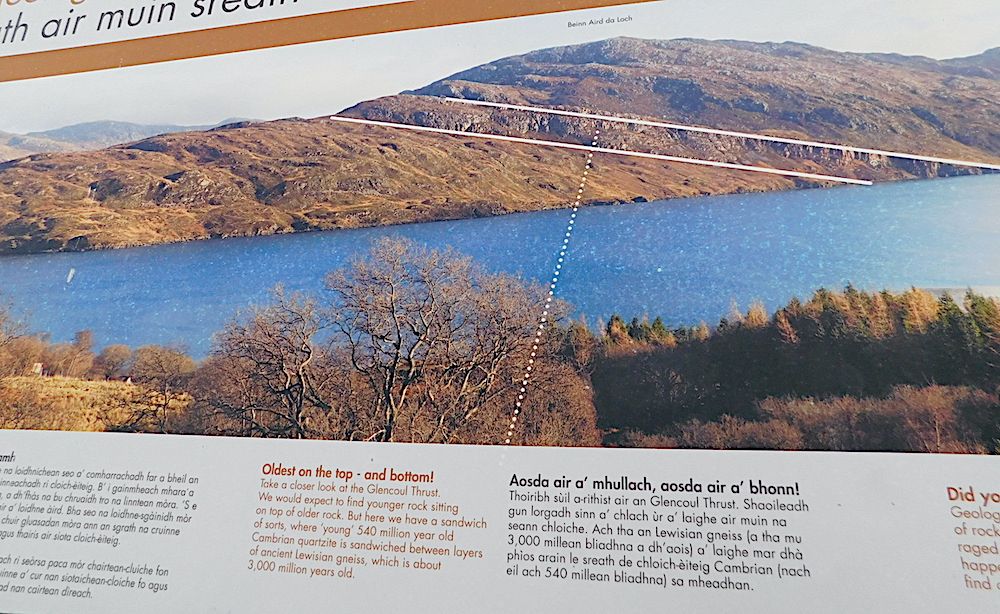
An information board about the Glencoul Thrust.
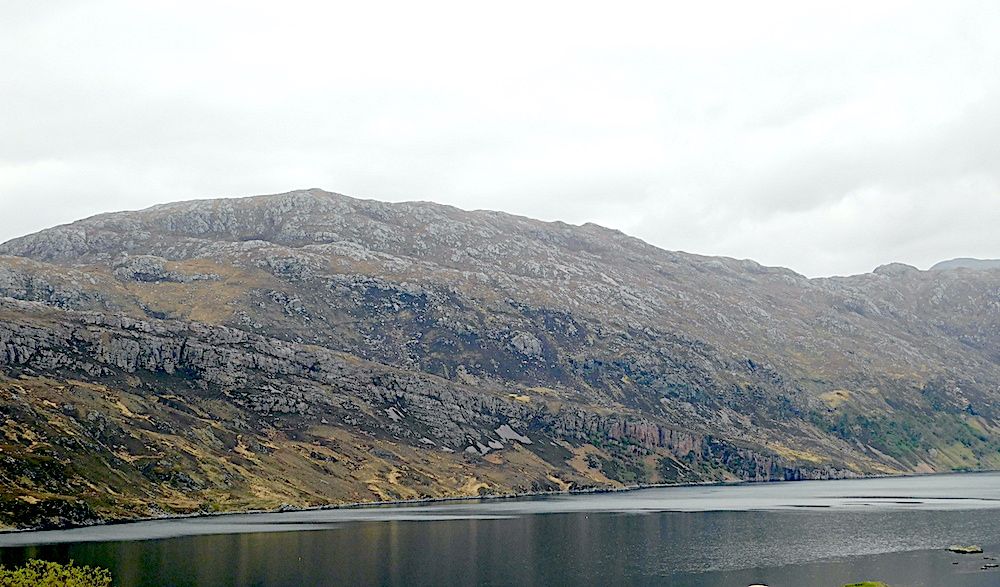
The Glencoul Thrust as seen on a cloudy day.
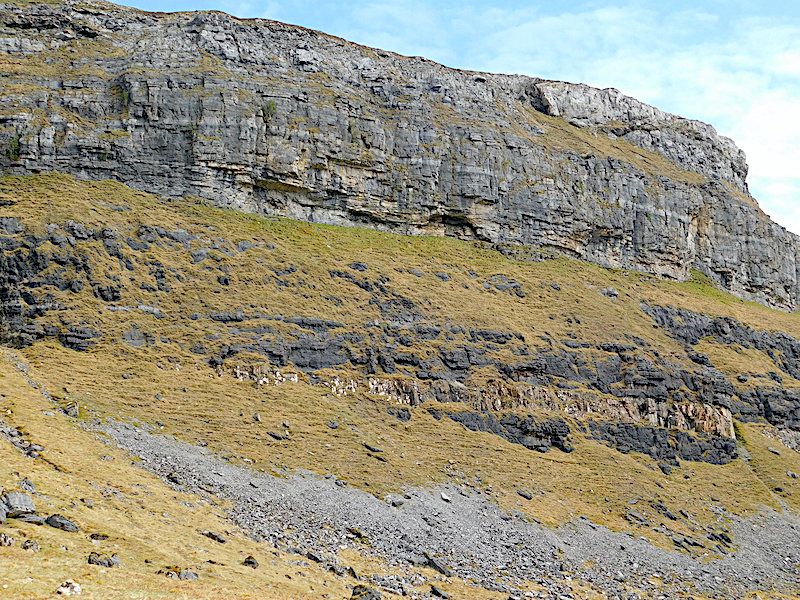
The chance sighting of a vogesite sill alongside the A837 a few miles South of Inchnadamph.
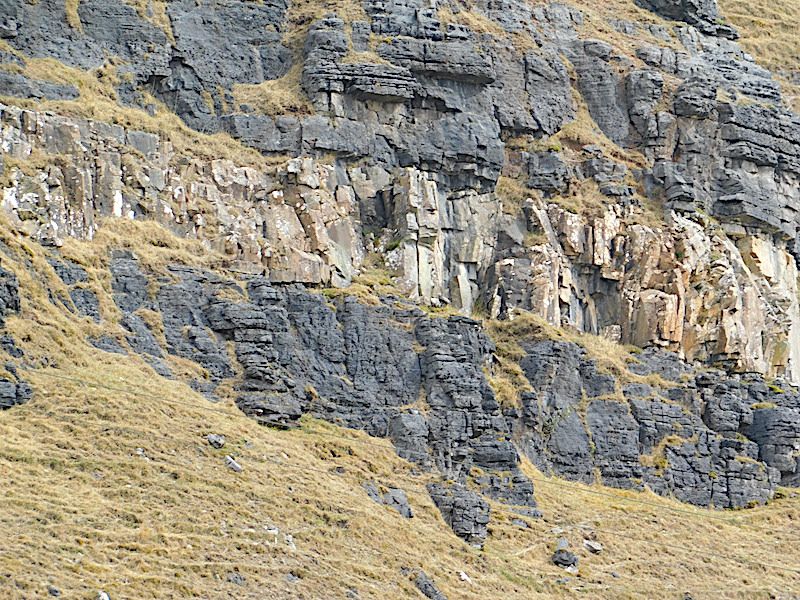
The vogesite sill intrudes the Ghrudaidh Formation – dolostones forming the base of the Durness limestones.
Vogesite is a plagioclase-rich lampropyre
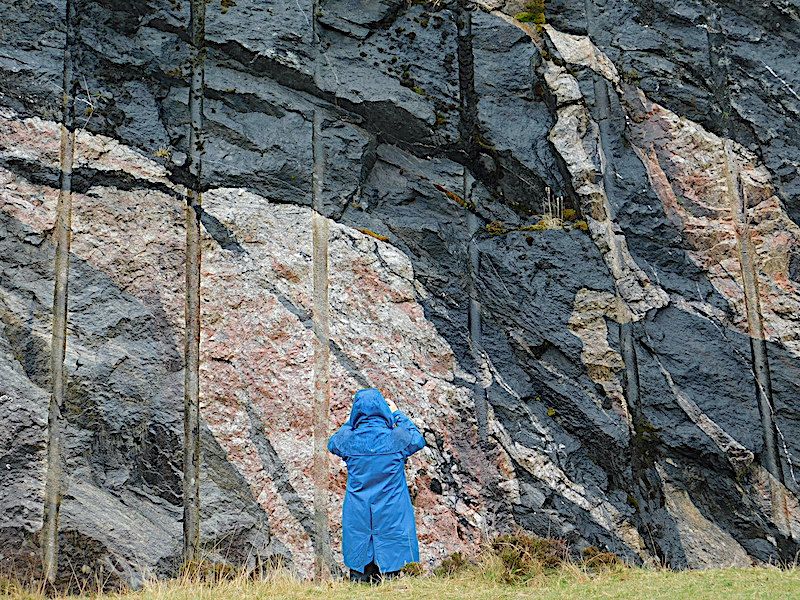
The “multi-coloured rock stop”. Three ages of rock intermingled. Gneiss around 2.8Ba, basaltic dykes around 2.3Ba and pegmatitic granites around 1.75Ba
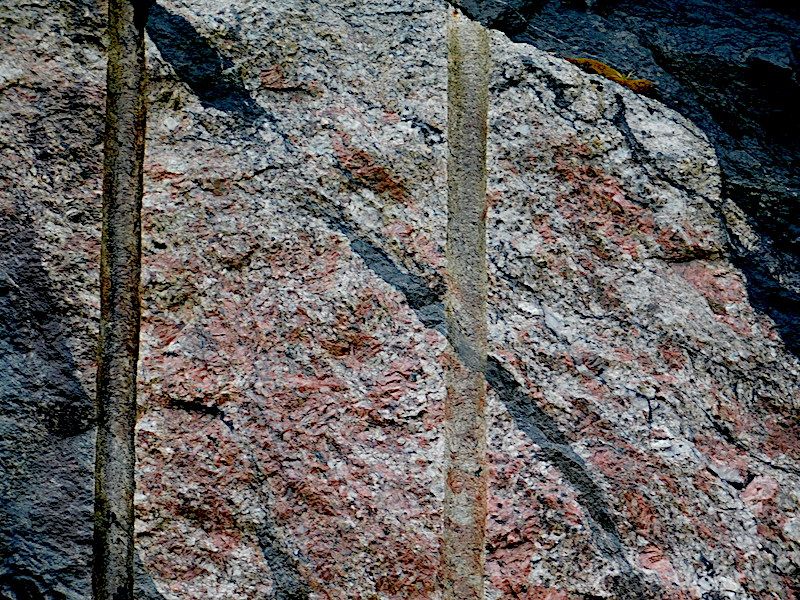
A close-up photograph of the pegmatitic granite -- Ignore the rock drill marks made when this road cutting was made. Nobody knew about this conjunction of rocks until the road was dug!
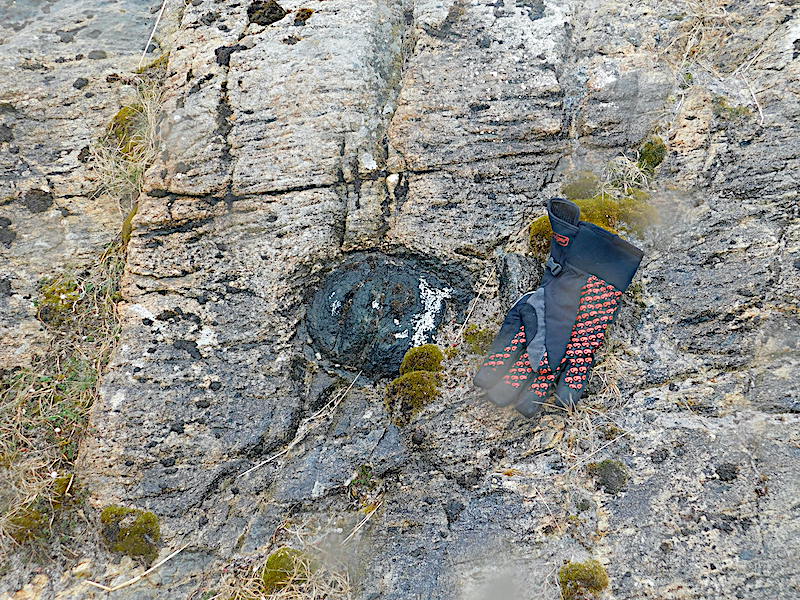
Achmelvich. Banded gneiss with olivine nodules.
The presence of these nodules suggests that the gneiss originated from a piece of oceanic crust
Thursday 13th July 2023
At the last meeting James Creswell of Geo World Travel https://www.geoworldtravel.com/ ) spoke about the geology of Namibia. His company leads many geological field trips every year with Namibia being one of them. The talk is based on a tour of Namibia commencing and finishing at Windhoek.
Namibia has a very varied geology and covers a vast time span. In fact the geology encompasses rocks of Archaean to Phanerozoic age and as such covers more than 2.6 billion years. About half of the country's surface area is bedrock exposure, while the remainder is covered by Cenozoic deposits of the Kalahari and Namib Deserts.
The north and south of the country were once two separate cratons which were once part of of the earlier super continent of Rodinia. Then during the Ediacaran and Cambrian periods ( 550-500Ma) the two collided during the Damara Orogeny. The resultant mountains have since eroded leaving central Namibia consisting of metamorphic inliers from the eroded roots of the mountains. The Damara Orogeny was actually part of a large Pan African Orogeny that created Gondwanaland. As the Cratons collided a thrust fault was created where older carbonate rocks slid as a ‘nappe’ over younger limestone rocks lubricated by a salty layer ( the Sole Dolomite). This is the Naukluft Thrust Fault and can be viewed in the Namib-Naukluft National Park.
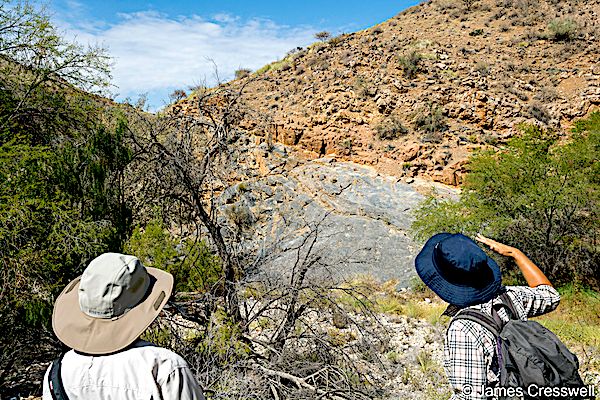
Nicole Grünert points to the Naukluft Thrust Fault
The oldest rocks to be seen are to be found at the Etosha national park and here you can find 2.2Ga paragneiss from the Congo Craton. Also in this area are drop-stones which fell out of ice-shelves or icebergs onto seafloor during one of the earth’s Snowball Earth episodes (630Ma). The Snowball Earth deposits themselves are covered by tropical carbonates known as Cap Carbonates. Other ancient rocks can be found near Kobos where there is an outcrop of Kalahari Craton basement granite which is 1Ga in age.
At Swakopmund basalt which formed as the super continent of Rodinia rifted apart was compressed and metamorphosed to amphibolite during the Pan-African Orogeny can be found as can rocks from the Matchless Belt which are thought to be an ophiolite.This belt lies between the Kalahari and Congo Cratons. Also at Swakopmund the world’s largest displayed quartz Crystal can be seen in the Crystal Gallery (Kristall Gallerie).
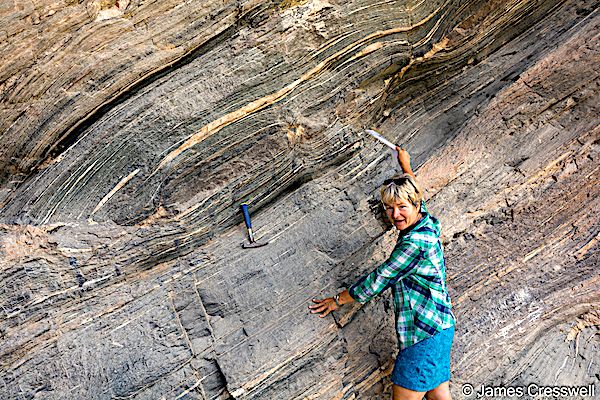
Nicole Grünert points to boudins in amphibolite which were once basalt dykes which later got metamorphosed in the Damara orogeny
As well as ancient rocks Namibia also boasts the presence of the oldest shelly fossils. These are the Namacalathus (549-542Ma) and their discovery caused the Precambrian (Edicarian)/Cambrian boundary to be revised back in time. These fossils are to be found in the area of the Tsaris Mountains.
The Erongo Complex is the eroded core of a huge volcano which has peripheral and central granite intrusions. Formed about 137-124Ma when Africa and South America were splitting apart to form the south Atlantic. The Erongo at 35km diameter is one of the largest Cretaceous granitic complexes in northern Namibia. It consists of both intrusive and extrusive rocks. The igneous activity gave rise to many different minerals which are sought after around the world. Similarly the Brandberg Massif ( Namibia’s highest mountain) is is a single mass of granite that pierced its way through the Earth’s crust into the Namib Desert during this time and is composed of homogeneous medium grained biotite-hornblende granite.
During the Carboniferous the whole of southern Africa was buried under a thick ice sheet. But during the Permian temperatures rose giving rise to an increase in fauna and flora. Now the remains of trees (Dadoxylon) can be found in rocks of the Lower Karoo Supergroup and is known as the “Petrified Forest” and has the biggest accumulation of these trees in southern Africa. They were deposited in an ancient river channel during flash flooding.
Jurassic sandstone may be found at the Waterberg Escarpment. This formed by the reactivation of a precambrian fault (Damaran) when Gondwana split to form the Atlantic. This caused Jurassic age Karoo sediments to be thrust over other Jurassic age sediments. Erosion of sediment has left the escarpment as seen today. The Waterberg Plateau is a popular national park.
One of Namibia’s great natural wonders is the Sossusvlei Pan and surrounding desert sand dunes. It is part of the Namib-Sand-Sea UNESCO site. The name translates to “dead-end marsh”. It is a clay and salt pan and is the terminus of the seasonal Tsauchab ephemeral river which is preventd from flowing all the way to the sea by the sand dunes of the Namib Desert. Some of the dunes are over 400m tall and are among the highest in the world. They are derived from material that eroded from the highlands of South Africa and Lesotho and was transported to the Namibian coast by rivers. This material was then blown onto land during the ice-age. The dunes have a red colour due to the grains of sand having a coating of iron oxide. In some places the dunes have a yellow colour due to the grains having a coating of clay particles from eroded local limestones.
What a truly remarkable place Namibia appears to be.
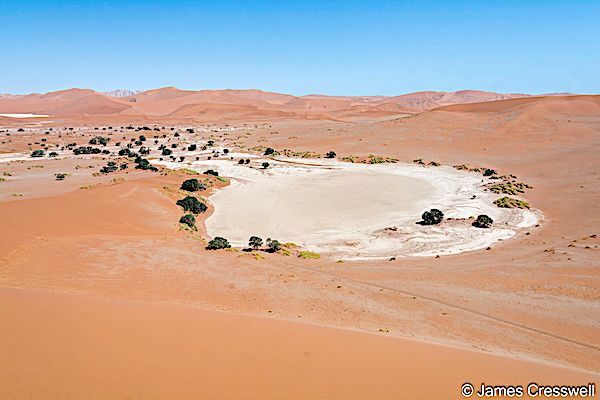
Deadvlei salt pan in the Namib Sand Sea UNESCO World Heritage Site