Thursday 4th November 2021
New data on the glaciations in Wales
At the last meeting Prof. Neil Glasser gave a highly informative talk regarding new data on the glaciations in Wales based on his recent research, in collaboration with Prof. Philip Hughes of Manchester University and Dr David Fink of the Australian Nuclear Science and Technology Organisation. (ANSTO).
Their research has been ongoing for a number of years and commenced by formulating a question regarding the thickness of ice over land in Wales during the ice-ages. Were the high elevations of Wales above the ice in the form of nunataks during this time or had they been covered by the ice? This led to the research project to determine exposure ages for parts of Wales which would determine if the high elevations had been covered by ice or not and would lead to a greater understanding of glacier retreat.
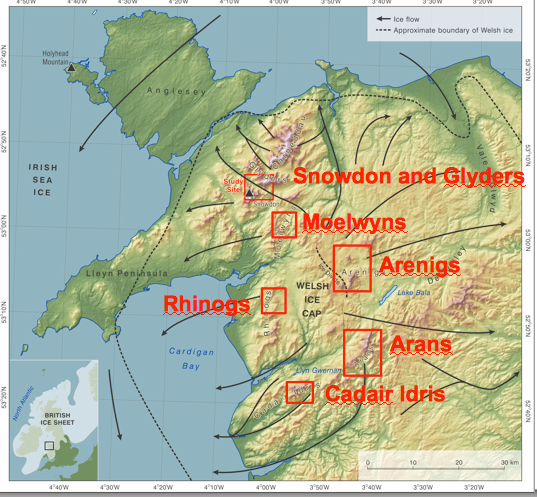
Research Area
The research area targeted seven major massifs in Wales with the aim of understanding the timing of glacier retreat from summits to cirques:
Moelwyns
Arenigs
Rhinogs
Arans
Snowdon and the Glyders
Cadair Idris
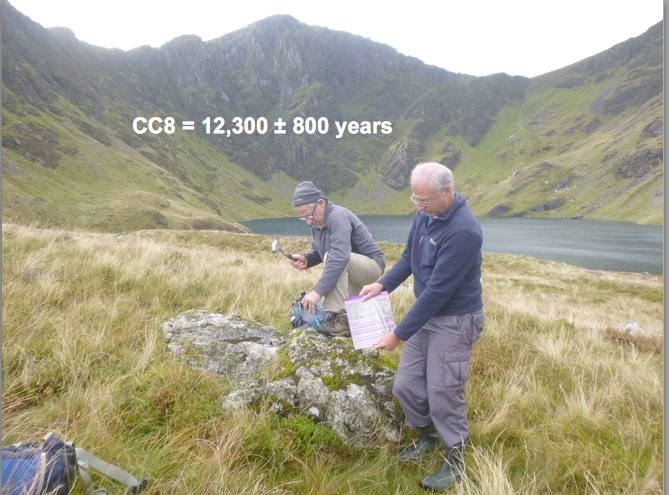
Taking Samples
"In order to determine the age at which the rocks were exposed they used the method of Cosmogenic Nucleotide Dating (CND). CND uses interactions between cosmic rays and nuclides in glacially transported boulders or glacially eroded bedrock to provide age estimates for rock exposure at the earth’s surface. That is how long the rocks have been located at the surface. Cosmogenic nuclide dating is effective over short to long timescales (1,000-10,000,000 years), depending on which isotope you are dating. Different isotopes are used for different lengths of times. This long period of applicability is an added advantage of cosmogenic nuclide dating."(http://antarticglaciers.org)
Sampling strategy is important as is choosing the correct rock type. Granite and sandstone boulders are frequently used as they contain a large amount of quartz which yields 10Be and 26Al via spallation of oxygen and silicon respectively. Using these paired cosmogenic isotopes produces more reliable results than when used in isolation.
Although Prof. Glasser touched on results from previous work on some of the targeted areas his talk presented us with the latest ( still unpublished) results from work carried out on Snowden and the Glyders and Cadair idris.
His previous work has shown that the summits of the Rhinogs, Moelwyns and Arenigs in north Wales were covered by ice when the last Welsh Ice Cap was at its maximum and evidence of ice scouring and transport of glacial boulders was found. The evidence was particularly pronounced in the Rhinogs and Moelwyns. This work also provided very strong evidence that these summits became exposed as nunataks at 20–19 ka.(Hughes et al.,2016)
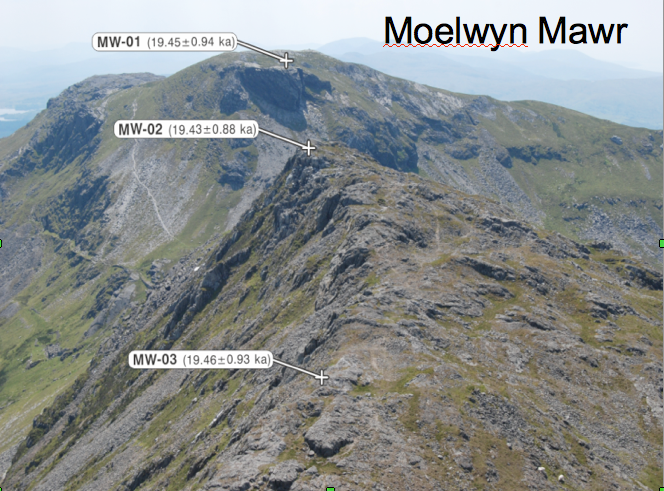
Other work on the Aran ridge showed that the welsh Ice Cap was thick enough to completely cover the Aran ridge and achieve glacial erosion at the Last Glacial Maximum. The work also showed that between 20 and 17,000 years ago the ridge summits were exposed as nunataks at a time when glacial erosion at lower elevations was achieved by large outlet glaciers in the valleys surrounding the mountains.( Glasser et al.,2012)
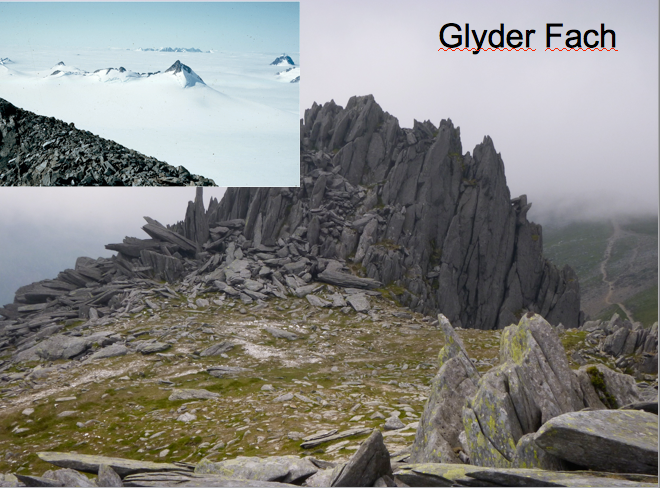
From the early works and the latest research on Snowdon and the Glyders and Cadair Idris the following conclusions have been derived:
Wales was glaciated by a large Ice cap that buried the highest mountains and transported erratics over the highest peaks in Marine Isotope Stage 4( MIS 4) 60-70,000 years ago. This latest research producing the first actual dates to show that this took place.
The highest summits of Wales then stood above the ice at LGM c 25,000 years ago.
Ice thinned quickly revealing most other summits 19-20,000 years ago after which there was only ice in the main valleys in Wales.
There was a pulse of glaciation at the Younger Dryas 12-10,000 years ago and ages for this have been obtained from both sides of Cadair Idris and also from Cwm Cau.
(Hughes PD, Glasser NF, Fink D, 2016. Rapid thinning of the Welsh Ice Cap at 20–19 ka based on 10Be ages. Quaternary Research 85: 107-117)
(Glasser NF, Hughes PD, Fenton C, Schnabel C, 2012.10Be and 26Al exposure-age dating of bedrock surfaces on the Aran ridge, Wales: evidence for a thick Welsh Ice Cap at the Last Glacial Maximum. Journal of Quaternary Science 27 (1): 97-104 )
Photos with kind permission of speaker
Friday 24th September 2021
At the last indoor (Zoom) meeting Prof. Sarah Davies ( Head of Geography and Environmental Sciences Aberystwyth University) gave a highly interesting talk entitled “Climate change and coastal heritage in Wales - insights from the palaeo-environmental record”
Prof. Davies is involved in a collaborative project known as CHERISH, i.e. Climate, Heritage and Environments of Reefs, Islands and Headlands. The works covers both Ireland and Wales and there are four partners:
Royal Commission on Historical Monuments of Wales, lead on the project.
Aberystwyth University
The Discovery programme - Centre for Archaeology and Innovation Ireland.
Geological Survey, Ireland.
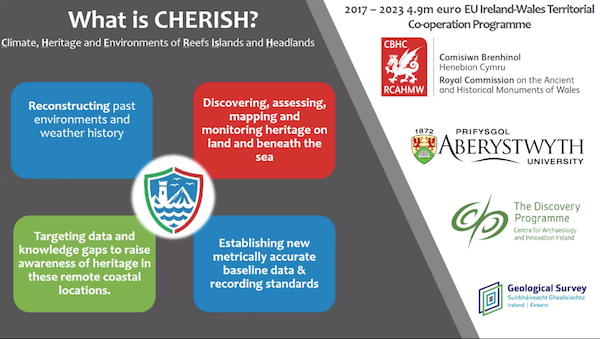
Using an array of techniques and a land, sea and air approach the project has the following aims:
Reconstructing past environments and weather history.
Discovering, assessing, mapping and monitoring heritage on land and beneath the sea.
Targeting data and knowledge gaps to raise awareness of heritage in these remote coastal locations.
Establishing new metrically accurate baseline data and recording standards.
Although the project covers both Ireland and Wales Prof. Davies focused her talk on Wales and the Holocene Period. Her team are focusing on trying to put some contemporary climate change problems into a longer term context i.e. the last few millennia. Using palaeoenvironmental records and a range of physical techniques they are examining coastal wetlands, lakes, bogs and sand dune systems, with the aim to date past storm activity and how periods of storminess have changed over time in frequency and intensity. The link to sea level change is also important.
The palaeoenvironmental evidence can also be used to link to the archaeology to provide environment and landscape context and how changes affected the societies at the time.
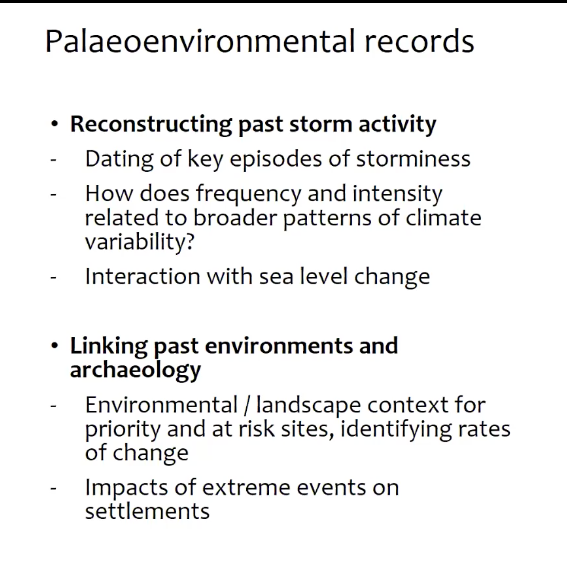
One study area covers parts of north Wales( Anglesey and Gwynedd) with different sites of different types and putting the data together from these sites it is hoped to develop a picture of change. These areas can be seen on the map below.
A key tool in the research is luminescence dating which allows dating of when minerals, especially quartz, was last exposed to light. In other words it is a burial signal for the minerals.
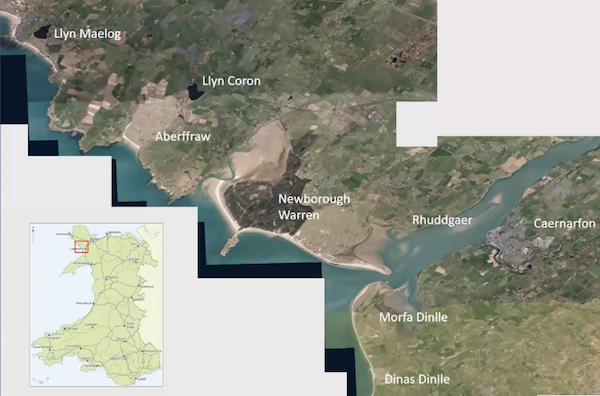
Prof. Davies then went on to describe the work undertaken at the Welsh sites. From the work undertaken a whole range of evidence has been obtained which is allowing them to build a picture of landscape and environmental change which can be integrated with the archaeology. This is aiding in the longer term understanding of environmental and climatic change and how past societies coped with those changes. This knowledge may help with the changes which we are undergoing now and in the future.
Photos with kind permission of speaker
Monday 16th August 2021
At the meeting on July 21st 2021 Dr Keith H.James (Honorary Fellow Geography and Earth Sciences, Aberystwyth University, Consultant Geologist) gave a very thought provoking talk entitled: The Problem of the Origin of the Carribean Plate in which he put forward his own model on it's origin.
Problems with Caribbean geology:
geology is spread over many different countries (fig.1)
some areas are poorly studied (access, vegetation, weathering, lack of interest)
there are no spreading ridges or calibrated magnetic anomalies (save for the centre of the Cayman Trough)
the plate interior is poorly known
there is no regional grid of modern, deep-looking seismic data
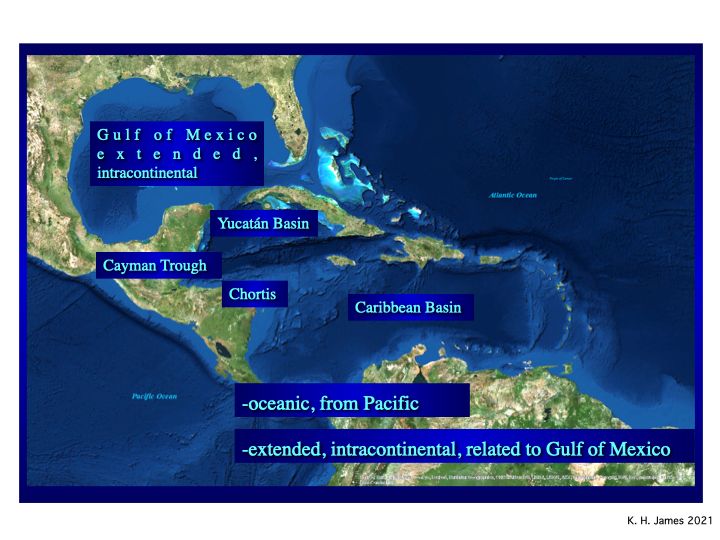
Fig.1
Caribbean geology is equivocal - there are two opposing models.
The dominant paradigm holds that the Caribbean Plate formed in the Pacific Ocean (e.g., Pindell & Kennan, 20091). It is therefore oceanic. It thickened to as much as 20 km, becoming the “Caribbean Large Igneous Province (CLIP) over the Galapagos “hotspot”. This collided with a roughly N-S trending, 1000 km long Pacific intra-oceanic arc, causing subduction polarity reversal, and drove the arc ahead of itself between N and S America (fig. 2). The arc became curved and extended to some 4000 km. As it collided with and accreted to N and S America it ceased activity. The Lesser Antilles arc is the active remnant of this arc. Chortis, a major continental block that forms the northwestern third of the Caribbean plate, rotated from SE Mexico to follow the CLIP into place.
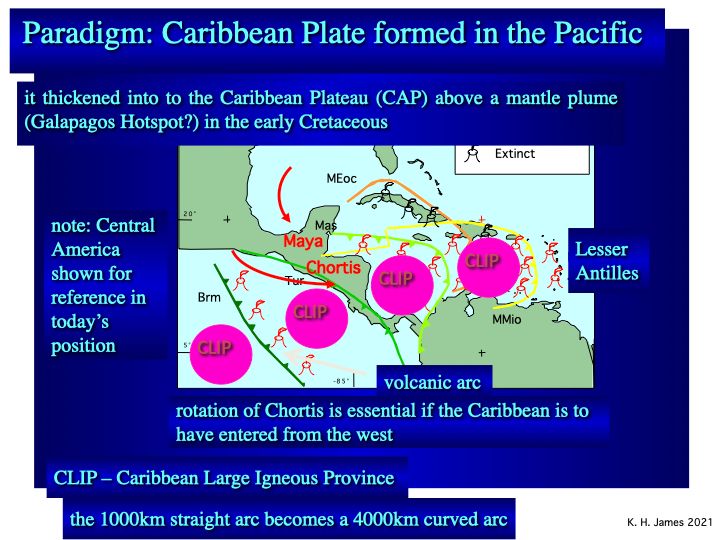
Fig.2
This model is incredibly complex1 and becomes increasingly so (4). It ignores data that deny it (2, 3). It prefaces most studies of the area, which begin with “it is well known that …..” and make no independent evaluation. There is no hydrocarbon potential.
It is impossible for a linear volcanic arc to become highly extended and curved. The segment supposedly accreted on Cuba alone is 1000 km long.
The CLIP is not a 20 km thick pile of basalt. DSDP drilling penetrated a few metres into the top of the “plateau”, calibrating a regional seismic Horizon B” as an upper Cretaceous dolerite.
The alternative is that the plate formed in place by intra-continental extension between N and S America (James, 2009, 2, 3). It shares history and geology with the Gulf of Mexico. This model is simple and accommodates all regional geology.
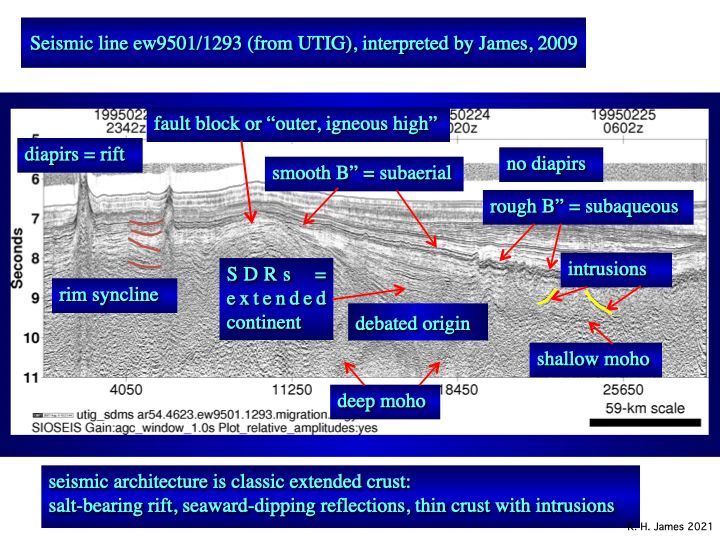
Fig.3
Chortis geology does not correlate with SE Mexico. The block manifests the regional tectonic trends of Middle America (James, 2002, fig. 3). It has not rotated. It has always been at the western end of the Caribbean and there was no gateway for the plate to enter.
Salt diapirs, misinterpreted as seamounts under the Pacific model, rise from below and lift Horizon B” (fig. 3). It is a single layer, not the top of a 20km thick pile. Thick, sub B” wedges (seaward-dipping reflectors or SDRs) repeat the geology of continental extension seen on Atlantic margins and in the Gulf of Mexico. They likely include Jurassic-Cretaceous carbonate reservoirs, similar to the very large sub-salt fields of Brazil´s offshore. The salt probably is Jurassic in age. Jurassic and Cretaceous source rocks are likely. There is outstanding hydrocarbon potential.
We know as much about the Caribbean-Yucatán basins today as we did about the North Sea in 1965. The basins are five times larger in area. While the Pacific model remains popular little progress will be made with understanding of the geology or the hydrocarbon potential.
References:
1 Pindell, J. and L. Kennan, 2009, Tectonic evolution of the Gulf of Mexico, Caribbean and northern South America in the mantle reference frame: an update: In: James, K. H. M. A. Lorente and J. Pindell, (Eds.), The Origin and Evolution of the Caribbean Plate, p. 1-55.
2 James, K. H., 2009a, In-situ origin of the Caribbean: discussion of data: In: James, K. H., M. A. Lorente and J. Pindell, (Eds.) Origin and evolution of the Caribbean Plate, Geological Society of London, Special Publications, v. 328, p. 77-125.
3 James, K. H., 2009b, Evolution of Middle America and the In-situ Caribbean Plate model: In: K. H. James, M. A. Lorente and J. Pindell, (eds.), Origin and evolution of the Caribbean Plate, Geological Society of London, Special Publications, v. 328, p. 127-138.
4 Pindell, J., D. Villagómez, R. Molina-Garza, R. Graham and B. Weber, 2020, A revised synthesis of the rift and drift history of the Gulf of Mexico and surrounding regions in the light of improved age dating of the Middle Jurassic salt: In: Davison, I. et al. (Eds.), The Basins, Orogens and Evolution of the Southern Gulf of Mexico and Northern Caribbean, GSL Special Publication 504, p. 29-76.
Text and photos with kind prmission of the speaker
Thursday 20th May 2021
At the meeting on 19th May Dr.Chris Simpson gave an illustated talk entitled: "Introduction to the geology of El Hierro." The talk was based on a guided trip round El Hierro organised by Chris Darmon at (http://www.geosupplies.co.uk) in 2019. El Hierro is the most Westerly of the Canary Islands, and one of the smallest. Its neighbour, Tenerife, is a well-known tourist destination with an international airport and a population around 900,000. El Hierro, by contrast, has only a small local airport and a population around 10,000. It is well off the tourist trail; and is generally only visited by people interested in the geology, the wildlife or for walking the footpaths.
Below is a simplified geology map of El Hierro
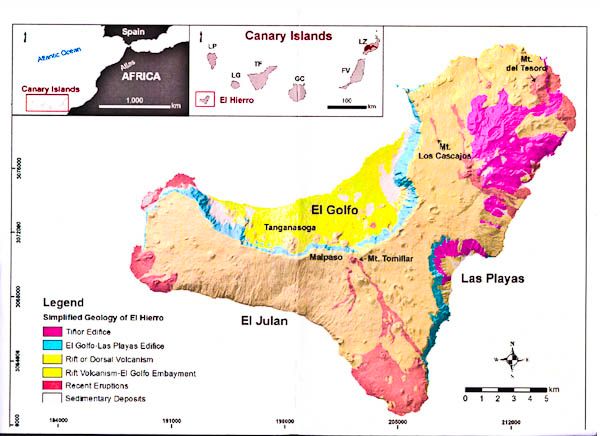
ResearchGate 2014 using Creative Commons Attribution 4.0 International
The two inset pictures give the location of the island. The main picture shows the tricorn shape of the island, which is typical of many volcanic islands because after the eruptions which lead to the formation of these islands, there are commonly episodes of flank collapse when large amounts of poorly consolidated rock fall away into the sea. El Golfo was the largest flank collapse when 120 cubic km of rock fell into the sea and produced a huge tsunami.
On the map, the darker red areas are the older volcanics (mainly on the NE corner) and the paler reds are the recent eruptions, mainly at the southern tip and the far west of the island.
The talk went through the variety of geological features which are well demonstrated in El Hierro and make it a worthwhile destination for any geologist: cinder cones, lava flows, ash deposits, dykes and the three impressive flank collapse vistas. The following selection of photographs gives a summary of what makes El Hierro special.
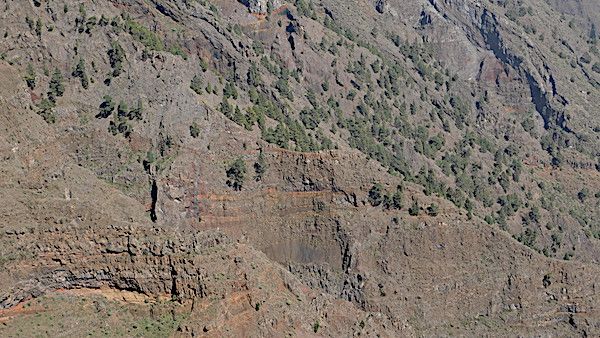
Mostly horizontal lava flows with some later flows at around 45° at the top right. Between some of the horizontal flows are reddish laterites – palaeosols
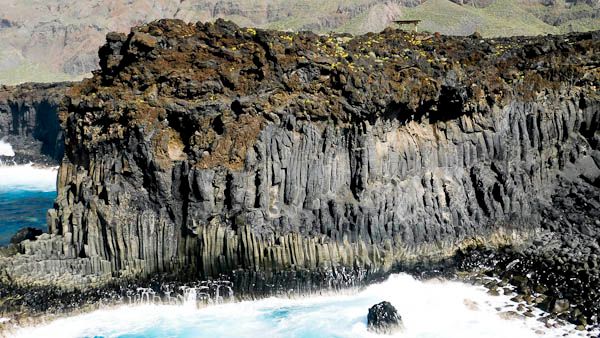
Successive lava flows with well-formed columnar jointing reminiscent of the Giant’s Causeway in Northern Ireland underneath. Along the top is a later flow of rubbly ‘A‘ā lava.
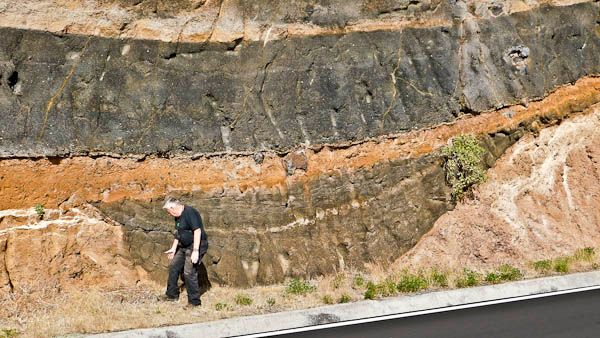
Successive layers of volcanic ash in a road cutting with our course leader, Chris Darmon, as the scale. Successive layers have very differing appearances; and this section includes by chance a channel-fill appearance
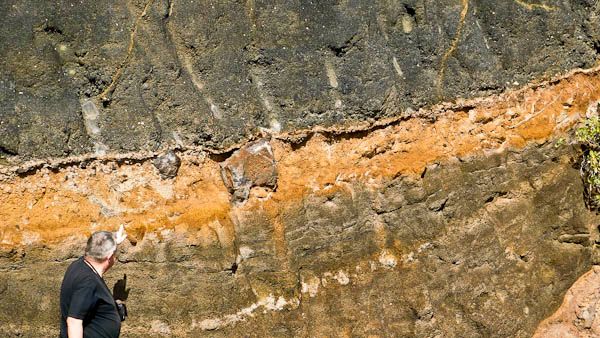
A higher power view showing some large volcanic bombs within otherwise fine ash deposits
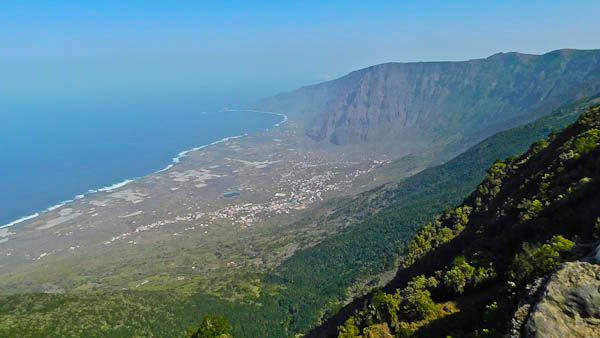
A view from the western flank of the El Golfo embayment – site of a huge flank collapse a few hundred thousand years ago. Numerous vertical dykes are seen in the near-vertical eastern wall. The flat land left after the collapse is now the main settlement on the island and the largest area for agriculture.
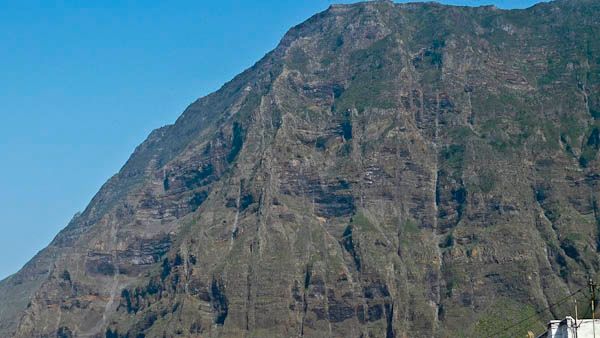
A higher-power view of the eastern flank. There are at least 12 vertical dykes visible in this small area, although they can be quite hard to discern at this magnification. The dykes cut across successive lava flows, so came after them. The existence of large numbers of dykes is one reason why flank collapse is so common on volcanic islands.
Friday 7th May 2021
The April meeting of the MWGC was at 7.15 p.m. on the 21st, when Ashley Patton, Engineering Geologist, BGS Cardiff, talked on Shallow Geothermal Energy in Cities.
This was our second talk on geothermal energy. Prof. Stimpson’s was concerned with deep geothermal, where the source is primordial or from radioactivity, whereas Ashley Patton’s was on ground sources nearer the surface. Harvesting such heat can contribute towards reducing reliance on traditional, increasingly insecure, CO2-producing thermal power generation.
A heat pump works by collecting heat from one place and transferring it to another against a temperature gradient.
Its performance is often measured by the “Coefficient of performance” or COP, the amount of useful heat put into a building divided by the amount of electrical energy used. It varies from 4 or 5 under favourable conditions to not much more than 1 if the heat source is very cold. Every degree is important.
Many domestic installations gather heat from shallow coils of pipe not much more than 1m below the surface, harvesting energy ultimately derived from solar, which has been stored in the soil.
BGS Cardiff have been researching a deeper source some tens of metres below ground where, particularly in urban areas, groundwater temperatures can be elevated owing to the “subsurface urban heat island” effect This is heat which has been accumulating over long periods of time, even decades, due to leakage from basements, industry and other human activities.
To establish feasibility and enable modelling, the “Cardiff Geo-Observatory” was set up, in which 3D temperature profiles were measured in a network of boreholes penetrating the sand and gravel aquifer below the city.
A pilot open-loop ground source system was also constructed at a school which operated satisfactorily and established feasibility as to what extent temperatures are depressed when heat is extracted.
Tapping this heat source could provide a useful proportion of the space heating necessary in areas of high housing density which often coincide with the presence of shallow aquifers and urban heat islands.
The talk was much appreciated as being both topical and one which will impact all our lives in the near future!